User:Wspaller/Physiology of antibiotic resistance in Mycobacterium tuberculosis
Introduction[edit]
Mycobacterium tuberculosis is one of the most important human pathogens in the world today. It is estimated that one third of the population is infected with TB and there are approximately 1.5 millions deaths in 2010(8). It is very difficult to treat with antibiotics and always requires treatment with 3-4 drugs simultaneously for 6-9 months.[1] A strain of Mycobacterium tuberculosis is multidrug resistant if it is resistant to at least the two major first line drugs, rifampicin and isoniazid.[2] Resistance occurs naturally but it is greatly accelerated by patients who do not finish the prescribed treatment, which as mentioned earlier generally lasts 6-9 months.[2] This article will cover the mechanisms and physiology Mycobacterium tuberculosis uses against a few of the most popular antibiotics.
![]() | |
Names | |
---|---|
IUPAC name
Systematic (IUPAC) name
5-(2,4-diguanidino- 3,5,6-trihydroxy-cyclohexoxy)- 4-[4,5-dihydroxy-6-(hydroxymethyl) -3-methylamino-tetrahydropyran-2-yl] oxy-3-hydroxy-2-methyl -tetrahydrofuran-3-carbaldehyde | |
Except where otherwise noted, data are given for materials in their standard state (at 25 °C [77 °F], 100 kPa).
|
Streptomycin[edit]
Streptomycin is a broad range antibiotic effective against several types of bacterial species, including active growing M. tuberculosis with MICs of 2-4 ug/ml. SM binds to the 30s subunit of the bacterial ribosome and inhibits protein synthesis by causing the mRNA to be misread during translation. Resistance to SM usually occurs by one of two different mutations and accounts for about 50% of resistant strains. The first being in the s12 protein which is encoded by rpsL gene and the second in the 16S rRNA encoded by the rss gene. The most common mutation that occurs in rpsL is a point mutation substituting arginine for lysine in the 43rd codon. This mutation confers high-level resistance. A second mutation in codon 88 is also common. Mutations in the 16S rRNA occur in the loops of the 16S rRNA. Several different point mutations are grouped around nucleotides 530 and 915 and result in an altered amino acid sequence conferring resistance.[3] These alterations cause low-level resistance (MICs 10ug/ml) and only play a minor role. [4] SM-resistant strains without mutations in rspL or rrs confer low-level resistance and account for 20%-30% of resistant strains. These strains occur by an array of different mutations such a mutation in gidB, which encodes a conserved methyltransferase specific for 16S rRNA.[3]
Isoniazid[edit]
One of the most extensively used first-line, anti-tuberculosis drugs currently used is isoniazid (INH).[3] INH affects only bacteria that are actively growing, and since Mycobacterium tuberculosis may be dormant for long periods of time, treatment of the bacteria with antimicrobial drugs must last for 6-12 months.[1] INH is activated when it interacts with KatG, a catalase- peroxidase enzyme that is encoded by the katG gene of the bacteria. When activated, INH produces reactive oxygen species and reactive organic species. These reactive species are thought to interact with NAD(H) (nicotinamide adenine dinucleotide), which forms an INHNAD complex. The main target of this complex is thought to be InhA enzyme, which plays a role in the elongation of fatty acids in mycolic acid synthesis. Resistance to INH can occur when M. tuberculosis loses or mutates the katG gene, and therefore reactive species are not made and mycolic acid synthesis continues as normal. This mechanism of resistance is generally seen in high-level resistant strains with a MIC (minimum inhibitory concentration) of >5 µg/ml. Other mechanisms of resistance to INH have been observed. Occasionally InhA is overexpressed due to a mutation in the promoter region of the mabA/inhA operon. A mutation can also occur at the active site of InhA so that the complex of reactive species and NAD(H) have less binding affinity to InhA, therefore causing less interruption of mycolic acid synthesis. This mechanism of resistance is generally seen in low-level resistant strains with a MIC of <1 µg/ml.[3]
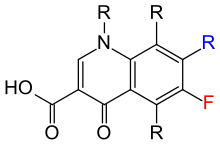


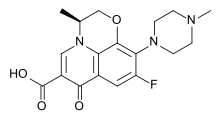

Quinolones[edit]
Quinolones are one of the most effective second-line drugs in the treatment of multidrug-resistant tuberculosis. M. tuberculosis develops resistance to quinolones following prolonged exposure, leading to the emergence of extensively drug-resistant (XDR) strains. This acquired resistance is due mainly to mutations in the DNA gyrase sequence.[5] Quinolones inhibit DNA gyrase, a type II toposimerase that is present in M. tuberculosis, resulting in microbial death. Topoisomerases regulate DNA supercoiling and nucleic acid tangling.[3] DNA gyrase is a tetrameric A2B2 protein. The A subunit carries the DNA breakage and reunion active site, whereas the B subunit promotes ATP hydrolysis. M. tuberculosis has gyrase A (gyrA) and gyrase B (gyrB) genes encoding the A and B subunits. Quinolone resistance in M. tuberculosis is predominantly due to mutations of the quinolone resistance-determining regions (QRDR) of gryA and gryB. Mutations in the QRDR of gryA have mainly been found clustered in codons 90, 91 and 94, with Ala-90 and Asp-94 being the most common. [6]A recent mechanism of quinolone resistance has been identified, which is mediated by MfpA. MfpA binds to DNA gyrase and inhibits its activity in the form of DNA mimcry. It’s expression causes the inhibitory effect on DNA gyrase and quinolone resistance.[3]
Rifampicin[edit]
Rifampicin is a an very important first line drug against Mycobacterium Tuberculosis and due to widespread use resistance is quite widespread. Rifampicin resistance is a result of the mutation in the rpoB gene, which encodes the RNA polymerase. In rifampicin susceptible strains, it will physically bind to the beta subunit of RNA polymerase just upstream of the catalytic center and prevent the elongation of the polypeptide. In resistant strains there are mutations present that do not allow for the binding of rifampicin, thus rendering it unable to kill the cell. The mutations in the amino acid chain of the RNA polymerase have been well characterized. A mutation from serine to leucine in the 531 position, histidine to arginine in the 526 position, and asparagine to valine in the 516 position are a few example of the mutations that result in high level resistance.[7] These mutations prevent rifampicin from binding the RNA polymerase and blocking the elongation of the growing polypeptide chain. Resistance to rifampicin occurs at frequency of 10^-7 to 10^-8.[1] Additionally there has even been rifampicin dependent strains observed, which exhibit good growth in the presence of rifampicin and poor growth in its absence.[1]
Pyrazinamide[edit]
Pyrazinamide is a first line drug that shortens the M. tuberculosis treatment from 9-12 months to 6 months called short term chemotherapy.[8] It is active only in vivo and kills the bacilli that are found in the lesions, where other drugs can not target. PZA (Pyrazinamide) works by first being hydrolyzed to pyrazinoic acid by the M. tuberculosis enzyme pyrazinamidase/ nicotinamidase. The pyrazinoic acid then undergoes passive diffusion to the cell surface along with an efflux pump. Once the pyrazinoic acid is outside of the cell, the acidic extracellular pH creates protonated uncharged pyrazinoic acid. This then diffuses back into the cell and accumulates, interrupting membrane potential and collapsing the proton motive force. The actual target of the pyrazinoic acid is unknown but it is linked with membrane energy metabolism. Fatty acid synthase I, was originally thought to be the target but has been discounted.[3] In most PZA- resistant M. tuberculosis strains, there is a mutation in the pncA gene, the gene that codes for the pyrazinamidase/nicotinamidase. Without this enzyme, pyrazinamide can not be activated to pyrazinoic acid and therefore can not go through with destroying the membrane potential of the cell. These mutations are highly diverse and can be found anywhere in the gene which is unique to PZA resistance. However, there is some degree of clustering at three regions of the PncA, 3–17, 61–85 and 132–142.[3] In other resistant strains such as pyrazinamidase-negative which have a high level of resistance, it is thought that there is a mutation in an undefined pncA regulatory gene. Another known strain with low level resistance has pyrazinamidase activity but no pncA mutations and its mechanism is still yet to be discovered.[3]
References[edit]
- ^ a b c d Gengenbacher, M. and Kaufmann, S. H.E. (2012), Mycobacterium tuberculosis: success through dormancy. FEMS Microbiology Reviews, 36: 514–532. doi: 10.1111/j.1574-6976.2012.00331.x
- ^ a b Centers for Disease Control and Prevention. Centers for Disease Control and Prevention, 17 Jan. 2012. Web. 01 May 2012. <http://www.cdc.gov/tb/topic/drtb/default.htm>
- ^ a b c d e f g h i Zhang Y, Yew WW. Mechanisms of drug resistance in Mycobacterium tuberculosis. International Journal of Tuberculosis and Lung Disease. 2009 Nov; 13(11):1320-30
- ^ Cooksey, Robert C., Glenn P. Morlock, Amy McQueen, Suzanne E. Glickman, and Jack T. Crawford. "Characterization of Streptomycin Resistance Mechanisms among Mycobacterium Tuberculosis Isolates from Patients in New York City." Ncbi May 1996. 1186-1188
- ^ Piton J, Petrella S, Delarue M, André-Leroux G, Jarlier V, et al. (2010) Structural Insights into the Quinolone Resistance Mechanism of Mycobacterium tuberculosis DNA Gyrase. PLoS ONE 5(8): e12245. doi:10.1371/journal.pone.0012245
- ^ Ricky W. T. Lau, Pak-Leung Ho, Richard Y. T. Kao, Wing-Wai Yew, et al. Molecular Characterization of Fluoroquinolone Resistance in Mycobacterium tuberculosis: Functional Analysis of gryA Mutation at Position 74. Antimicrobial Agents and Chemotherapy. 2011; 55:608-614
- ^ Williams, D. L., C. Waguespack, K. Eisenach, and J. T. Crawford. "Characterization of Rifampin-resistance in Pathogenic Mycobateria." Antimicrobial Agents and Chemotherapy 38.10 (1994). Print.
- ^ Shi, W., X. Zhang, X. Jiang, H. Yuan, J. S. Lee, C. E. Barry, H. Wang, W. Zhang, and Y. Zhang. "Pyrazinamide Inhibits Trans-Translation in Mycobacterium Tuberculosis." Science 333.6049 (2011): 1630-632. Print